Spectrometers are technology for reading light that date back to the era of famed 17th-century physicist Isaac Newton. They work by breaking down light waves into their different colors — or spectra — to provide information about the makeup of the objects being measured.
UC Santa Cruz researchers are designing new ways to make spectrometers that are ultra-small but still very powerful, to be used for anything from detecting disease to observing stars in distant galaxies. Their inexpensive production cost makes them more accessible and customizable for specific applications.
The team of researchers, led by an interdisciplinary collaboration between UC Santa Cruz Professor of Electrical and Computer Engineering Holger Schmidt and Professor of Astronomy and Astrophysics Kevin Bundy, published the details of their device in a paper in APL Photonics, a premier journal in the field.
The researchers demonstrate a novel, extremely high-performance spectrometer that can measure light with a 0.05 nanometers wavelength resolution. That’s about 1.6 million times smaller than the width of a human hair, and the same resolution that can be achieved on a device 1,000 times bigger.
“That's essentially as good as a big, standard, expensive spectrometer,” said Schmidt, the senior author on the paper and a long-time expert in developing chips for light detection. “That’s really pretty impressive and very competitive.”
Miniature devices
Miniaturizing spectrometers is an active area of research, as spectrometers are used in many fields but can be as big as a three-story building and extremely expensive. However, miniaturized spectrometers often do not perform as well as bigger instruments, or they are very difficult and expensive to manufacture because they require extremely precise nanofabrication.
UC Santa Cruz researchers have created a device that is able to achieve high performance without such costly manufacturing. Their device is a miniature, high-powered waveguide which is mounted on a chip and used to guide light into a specific pattern, depending on its color.
Information from the chip is fed into a machine learning algorithm that reads the patterns created by different wavelengths of light in order to reconstruct the image with extremely high accuracy and precision — an approach is called “reconstructive” spectrometry.
This technique produces accurate results because the machine learning algorithms don’t require highly precise input to be able to distinguish the light patterns, and can constantly improve upon their own performance and optimize themselves to the hardware.
Because of this, the researchers can make the chips with relatively easy and inexpensive fabrication techniques, in a process that takes hours rather than weeks. The lightweight, compact chips for this project were designed at UCSC, and fabricated and optimized at Brigham Young University in partnership with Schmidt’s longtime collaborator Professor Aaron Hawkins and his undergraduate students.
“Compared to more sophisticated chip design, this only requires one photolithography mask which makes the fabrication much easier and much faster,” Hawkins said. “Someone with some basic capabilities could reproduce this and create a similar device tuned to their own needs.”
Reading the stars
The researchers envision that this technology can be used for a wide range of applications, though their preliminary focus is to create powerful instruments for astronomy research. Because their devices are relatively inexpensive, astronomers could specialize them to their specific research interests, which is practically impossible on much larger instruments that cost millions of dollars.
The research team is working to make the chips functional on the UC-operated Lick Observatory telescope, first to take in light from a star and later to study other astronomical events. With such high accuracy on these devices, astronomers could start to understand phenomena such as the makeup of atmospheres on exoplanets, or probing the nature of dark matter in faint dwarf galaxies. The comparatively low cost of these devices would make it easier for scientists to optimize them for their specific research interests, something nearly impossible on traditional devices.
Leveraging long standing expertise at UC Santa Cruz in adaptive optics systems for astronomy, the researchers are collaborating to figure out how to best capture the faint glimmers of light from distant stars and galaxies and feed it through into the miniaturized spectrometer.
“In astronomy, when you try to put something on a telescope and get light through it, you always discover new challenges — it’s much harder than just doing it in the lab. The beauty of this collaboration is that we actually have a telescope, and we can try deploying these devices on the telescope with a good adaptive optics system,” Bundy said.
Uses for health and beyond
Beyond astronomy, the research team shows in this paper that the tool is capable of fluorescence detection, which is a noninvasive imaging technique used for many medical applications, such as cancer screening and infectious disease detection.
In the future, they plan to develop the technology for Raman scattering analysis. This is a technique that uses light scattering for the detection of any unique molecule, often used as a specialized test to look for a specific chemical substance, such as the presence of drugs in the human body or toxic pollutants in the environment. Because the system is so straightforward and does not require the use of heavy instrumentation or fluidics like other techniques, it would be convenient and robust for use in the field.
The researchers also demonstrate that the compact waveguides can be placed alongside each other to enhance the performance of the system, as each chip can measure a different spectra and provide more information about whatever light it is observing. In the paper the researchers demonstrate the power of four waveguides working together, but Schmidt envisions that hundreds of chips could be used at once.
This is the first device shown to be able to use multiple chips at once in this way. The researchers will continue to work to improve the sensitivity of the device to get even higher spectral resolution.
Ultra-small spectrometer yields the power of a 1,000 times bigger device
The tiny, relatively inexpensive devices could be used for customized astronomy research
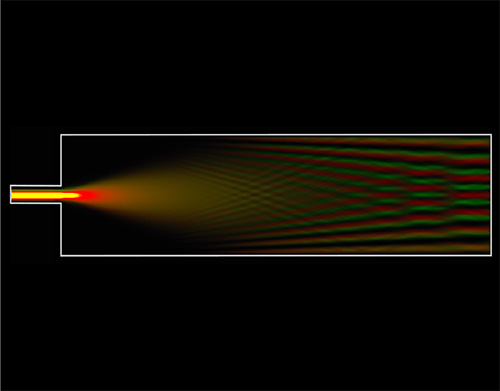
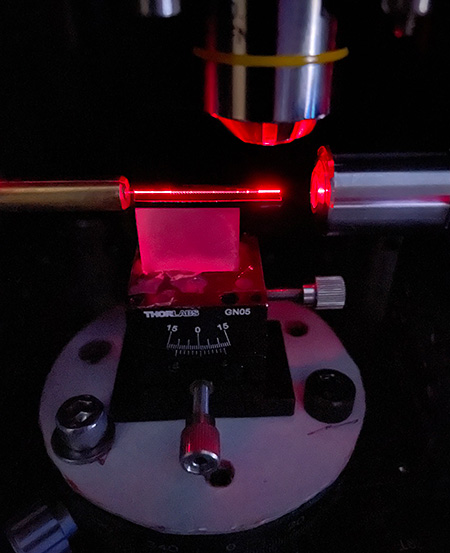