Giant planets in our solar system and circling other stars have exotic clouds unlike anything on Earth, and the gas giants orbiting close to their stars—so called hot Jupiters—boast the most extreme.
A team of astronomers from the United States, Canada and the United Kingdom have now come up with a model that predicts which of the many types of proposed clouds, from sapphire to smoggy methane haze, to expect on hot Jupiters of different temperatures, up to thousands of degrees Kelvin.
Surprisingly, the most common type of cloud, expected over a large range of temperatures, should consist of liquid or solid droplets of silicon and oxygen, like melted quartz or molten sand. On cooler hot Jupiters, below about 950 Kelvin (1,250 degrees Fahrenheit), skies are dominated by a hydrocarbon haze, essentially smog.
The model will help astronomers studying the gases in the atmospheres of these strange and distant worlds, since clouds interfere with measurements of the atmospheric composition. It could also help planetary scientists understand the atmospheres of cooler giant planets and their moons, such as Jupiter and Saturn’s moon Titan in our own solar system.
“In studying planetary atmospheres in the solar system, we typically have the context of images. We have no such luck with exoplanets. They are just dots or shadows,” said Jonathan Fortney, professor of astronomy and astrophysics at UC Santa Cruz. “That's a huge loss in information. But what we do have to make up for that is a much larger sample size. And that allows us to look for trends—here, a trend in cloudiness—with planetary temperature, something that we just don't have the luxury of in our solar system.”
Fortney and others at UCSC, UC Berkeley, and other institutions have been working on the model for the past two years. Peter Gao, a postdoctoral fellow now at UC Berkeley who will be joining Fortney at UCSC later this year, is first author of a paper describing the model published May 25 in the journal Nature Astronomy.
“The kinds of clouds that can exist in these hot atmospheres are things that we don’t really think of as clouds in the solar system,” Gao said. “There have been models that predict various compositions, but the point of this study was to assess which of these compositions actually matter and compare the model to the available data that we have.”
The study takes advantage of a boom in the study of exoplanet atmospheres over the past decade. Though exoplanets are too distant and dim to be visible, many telescopes, in particular the Hubble Space Telescope, are able to focus on stars and capture starlight passing through the atmospheres of planets as they pass in front of their star. The wavelengths of light that are absorbed, revealed by spectroscopic measurements, tell astronomers which elements make up the atmosphere. To date, this technique and others have found or inferred the presence of water, methane, carbon monoxide and carbon dioxide, potassium and sodium gases and, in the hottest of the planets, vaporized aluminum oxide, iron and titanium.
But while some planets seem to have clear atmospheres and clear spectroscopic features, many have clouds that completely block the starlight filtering through, preventing the study of gases below the upper cloud layers. The composition of the gas can tell astronomers how exoplanets form and whether the building blocks of life are present around other stars.
“We have found a lot of clouds: some kinds of particles—not molecules, but small droplets—that are hanging out in these atmospheres,” Gao said. “We don't really know what they are made of, but they are contaminating our observations, essentially making it more difficult for us to assess the composition and abundances of important molecules like water and methane.”
Ruby clouds
Astronomers have proposed many strange types of clouds to explain these observations, composed of aluminum oxides, such as corundum, the stuff of rubies and sapphires; molten salt, such as potassium chloride; silicon oxides, or silicates, like quartz, the main component of sand; sulfides of manganese or zinc that exist as rocks on Earth; and organic hydrocarbon compounds. They could be liquid or solid aerosols, Gao said.
Gao adapted computer models initially created for Earth’s water clouds, and subsequently extended to the cloudy atmospheres of planets like Jupiter, which has ammonia and methane clouds. He expanded the model even further to the much higher temperatures seen on hot gas giant planets—up to 2,800 Kelvin, or 4,600 degrees Fahrenheit (2,500 degrees Celsius)—and the elements likely to condense into clouds at these temperatures.
The model takes into account how gases of various atoms or molecules condense into a droplet, how the droplets grow or evaporate and whether they are likely to be transported in the atmosphere by winds or updrafts or sink because of gravity.
“The idea is that the same physical principles guide the formation of all types of clouds,” said Gao, who has also modeled sulfuric acid clouds on Venus. “What I have done is to take this model and bring it out to the rest of the galaxy, making it able to simulate silicate clouds and iron clouds and salt clouds.”
He then compared his predictions to available data on 30 exoplanets, out of a total of about 70 transiting exoplanets with recorded transmission spectra to date.
The model revealed that many of the exotic clouds proposed over the years are difficult to form because the energy required to condense is too high. Silicate clouds condense easily, however, and dominate over a 1,200-degree Kelvin range of temperatures: from about 900 to 2,000 Kelvin. That’s a range of about 2,000 degrees Fahrenheit.
According to the model, in the hottest atmospheres, aluminum oxides and titanium oxides condense into high-level clouds. In exoplanets with cooler atmospheres, those clouds form deeper in the planet and are obscured by higher silicate clouds. On even cooler exoplanets, these silicate clouds also form deeper, leaving clear upper atmospheres. At even cooler temperatures, ultraviolet light from the star converts organic molecules like methane into extremely long hydrocarbon chains that form a high-level haze akin to smog. This smog can obscure lower-lying salt clouds of potassium or sodium chloride.
For those astronomers seeking a cloudless planet to more easily study the gases in the atmosphere, Gao suggested focusing on planets between about 900 and 1,400 Kelvin, or those hotter than about 2,200 Kelvin.
“The presence of clouds has been measured in a number of exoplanet atmospheres before, but it is when we look collectively at a large sample that we can pick apart the physics and chemistry in the atmospheres of these worlds,” said co-author Hannah Wakeford, an astrophysicist at the University of Bristol in the U.K. “The dominant cloud species is as common as sand—it is essentially sand—and it will be really exciting to be able to measure the spectral signatures of the clouds themselves for the first time with the upcoming James Webb Space Telescope.”
Future observations, such as those by NASA’s JWST, scheduled for launch within a few years, should be able to confirm these predictions, and perhaps shed light on the hidden cloud layers of planets closer to home. Gao said that similar exotic clouds may exist at depths within Jupiter or Saturn where the temperatures are close to those found on hot Jupiters.
“Because there are thousands of exoplanets versus just one Jupiter, we can study a bunch of them and see what the average is and how that compares to Jupiter,” Gao said.
He and his colleagues plan to test the model against observational data from other exoplanets and also brown dwarfs, which are basically gas giant planets so massive they’re almost a star. They, too, have clouds.
Other co-authors of the paper are Daniel Thorngren of the University of Montreal in Canada, Graham Lee of Oxford University in the U.K., Diana Powell and Xi Zhang of UC Santa Cruz, Caroline Morley of the University of Texas at Austin, and Kevin Stevenson of the Applied Physics Laboratory at Johns Hopkins University in Maryland.
Gao was supported by NASA’s postdoctoral program and a 51 Pegasi Fellowship from the Heising-Simons Foundation.
Astronomers create cloud atlas for hot, Jupiter-like exoplanets
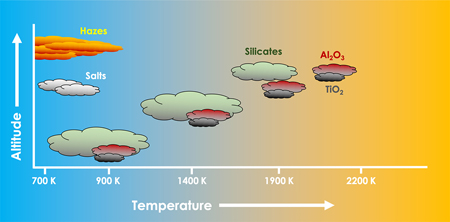